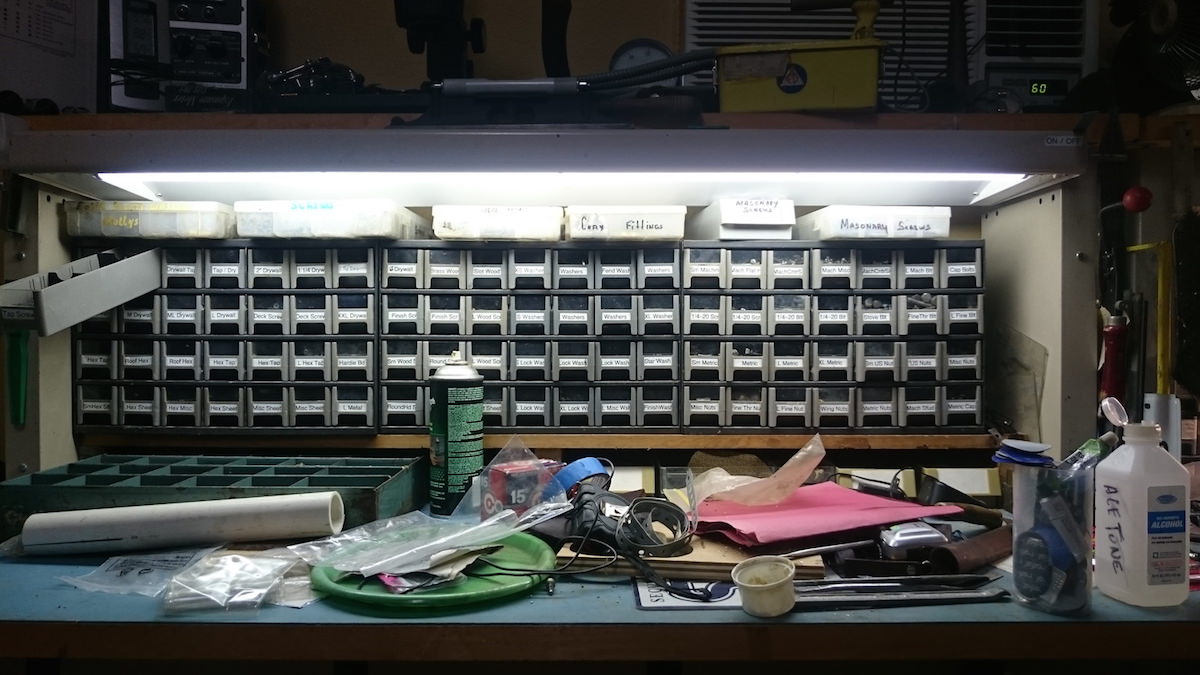
NOTES AND NEWS
Reciprocity: vibration isolation works the same, regardless of which way you look
Everything has a natural frequency: the structural floor, the lab bench, the vibration-isolated system. Even the microscope itself has internal resonances; these are the reason why the instrument is sensitive to vibrations in the first place. And when it comes to vibration isolation, allowing these resonances line up (in frequency) is usually not what we want.
Machine vibration isolation failures
Part of what's vexing for machine isolation is the sheer number of options, and the fact that machine vibration impacts evolve over time. In contrast to the structural vibration design (for which there are only so many kinds of steel and concrete materials, concepts, and techniques), machine vibration isolation is heavily product-driven and sensitive to installation variability. And while that structure doesn't much change over the years, rotating machinery encounters wear-and-tear while isolators don't always stay in alignment.
Why vibration isolation frequency matters
When your vibration consultant tells you that he or she really thinks you should use springs instead of neoprene pads for something, there's probably a reason! Frequency matters, and it's actually possible for the wrong kind of "isolator" to make building vibrations worse instead of better.
We need a better word than "isolator"
Most of our projects depend on liberal application of vibration isolation systems on mechanical equipment. Especially in nanotech labs and other high-tech settings, you simply can't throw enough concrete and steel at the problem. It's far better -- and far cheaper -- to just minimize the vibrational forces that get applied to the structure in the first place. But it bears repeating: resilient-support isolation systems can't eliminate vibrations. At best, they can only only reduce vibrations. Critically: the effectiveness of vibration isolators depends on frequency.