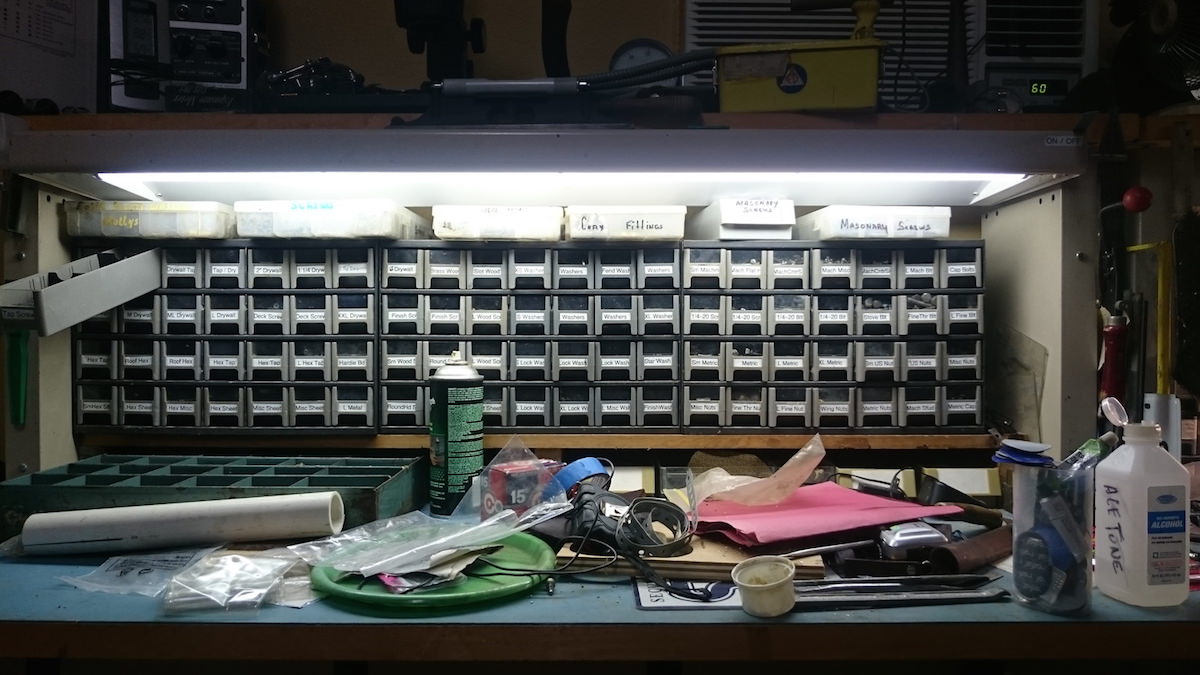
NOTES AND NEWS
The economic argument for transit system vibration mitigation
Good policy means finding ways to economically introduce mass transit access for R&D campuses and surrounding communities without damaging or limiting the research functions at the core of the institutional mission.
What kinds of building vibrations are relevant to labs?
It should be obvious that lab tools are generally more sensitive than people. But it might not be obvious just how sensitive the different tools and processes are. In this blog post we put some “order-of-magnitude” numbers on these vibration sensitivities and typical levels.
Traffic vibration: road surface quality drives vibration impacts
Just how much vibration impact can traffic create? Vibrasure produced a video at a large research institution in North America to show how significant it can be in academic laboratories.
Building vibration: mechanical systems vibration isolation
Machine vibration isolation schemes fail for all kinds of reasons, ranging from conceptual problems to bad hardware selections to poor installation. So, how can you tell if mechanical systems' isolators are working? A formal test is expensive and requires a lot of planning and coordination. Here, Vibrasure has produced a video on a easy way to tell if the isolators are working.
Environmental vs local sources of building vibration
Floor vibrations in labs aren’t uniform across the spectrum. But what governs how much energy we see at different frequencies in the spectrum? Different kinds of sources contribute to or even dominate different parts of the spectrum. The first and most obvious distinction between different "kinds of sources" is local vs. environmental. What is interesting about this differentiation is the degree of control that might be exerted over those sources.
Can we isolate this microscope from floor vibrations?
For projects that house sensitive instruments and activities – like nanotech labs or vivariums – vibration and noise impacts from the outside world can interfere with research productivity. Since there's only so much you can do about the environment, we are often asked about local vibration isolation systems that act right at the tools themselves. From a technical perspective, the biggest thing to keep in mind is that these isolation schemes can only attenuate -- not eliminate -- floor vibrations.
Reciprocity: vibration isolation works the same, regardless of which way you look
Everything has a natural frequency: the structural floor, the lab bench, the vibration-isolated system. Even the microscope itself has internal resonances; these are the reason why the instrument is sensitive to vibrations in the first place. And when it comes to vibration isolation, allowing these resonances line up (in frequency) is usually not what we want.