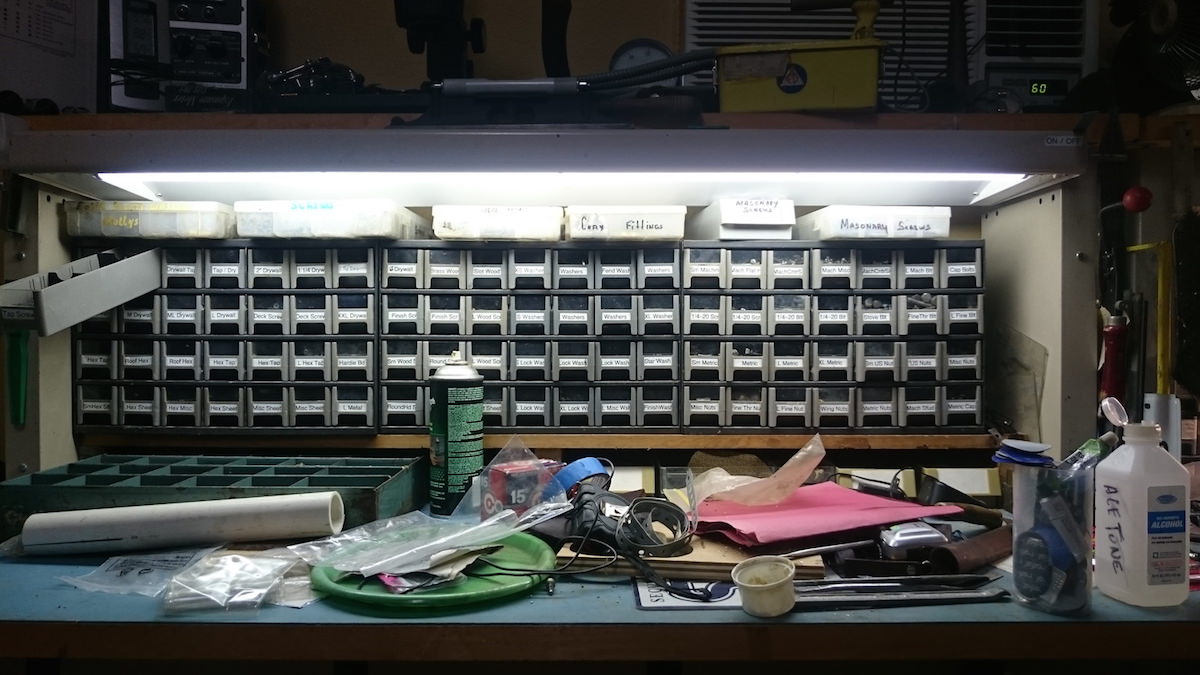
NOTES AND NEWS
Environmental vs local sources of building vibration
Floor vibrations in labs aren’t uniform across the spectrum. But what governs how much energy we see at different frequencies in the spectrum? Different kinds of sources contribute to or even dominate different parts of the spectrum. The first and most obvious distinction between different "kinds of sources" is local vs. environmental. What is interesting about this differentiation is the degree of control that might be exerted over those sources.
Can we isolate this microscope from floor vibrations?
For projects that house sensitive instruments and activities – like nanotech labs or vivariums – vibration and noise impacts from the outside world can interfere with research productivity. Since there's only so much you can do about the environment, we are often asked about local vibration isolation systems that act right at the tools themselves. From a technical perspective, the biggest thing to keep in mind is that these isolation schemes can only attenuate -- not eliminate -- floor vibrations.
How to Read Centile Statistical Vibration Data
For field or building-wide surveys, our practice is to supplement the spatial data gathered across the site with data from (at least) one location gathered over time. This really helps illustrate how much of the observed variability in the spatial data might actually be due to temporal variability. But interpreting these data isn't intuitive for some people.
How to think about footfall vibration from walkers in buildings
Even in buildings with cutting-edge imaging suites, there’s often tens or hundreds of square feet of laboratory and office space for every square foot of basement-level SEM/TEM Room space. That means that our job isn’t finished when we’ve made the electron microscopes and scanning probes happy; we still need to make everyone upstairs comfortable and productive, too.
Temporal variability in vibration environments
Timescale is an important parameter in considering vibration impacts. And while there are technical reasons to consider timescale (is my apparatus even sensitive to milli-second-scale excursions? what are the chances that I'm even doing something sensitive at the moment when the transient occurs?) economic and practical considerations can be just as important. If your lab executes experiments that take huge budgets and months of planning to pull off, then even rare events might be a real threat, if only because the consequences of failure (however unlikely) are so dire.
A quick note regarding vibration and noise units
Just a quick note regarding expressions of vibration and acoustical data. Every now and then we come upon a vexing problem related to full expressions of the units of a measurement (or criterion). I'm not talking about gross errors, like confusion of "inches-vs-centimeters" or "pounds-vs-newtons". Instead, I'm referring to some of the other, more subtle parts of the expression, like scaling and bandwidth.